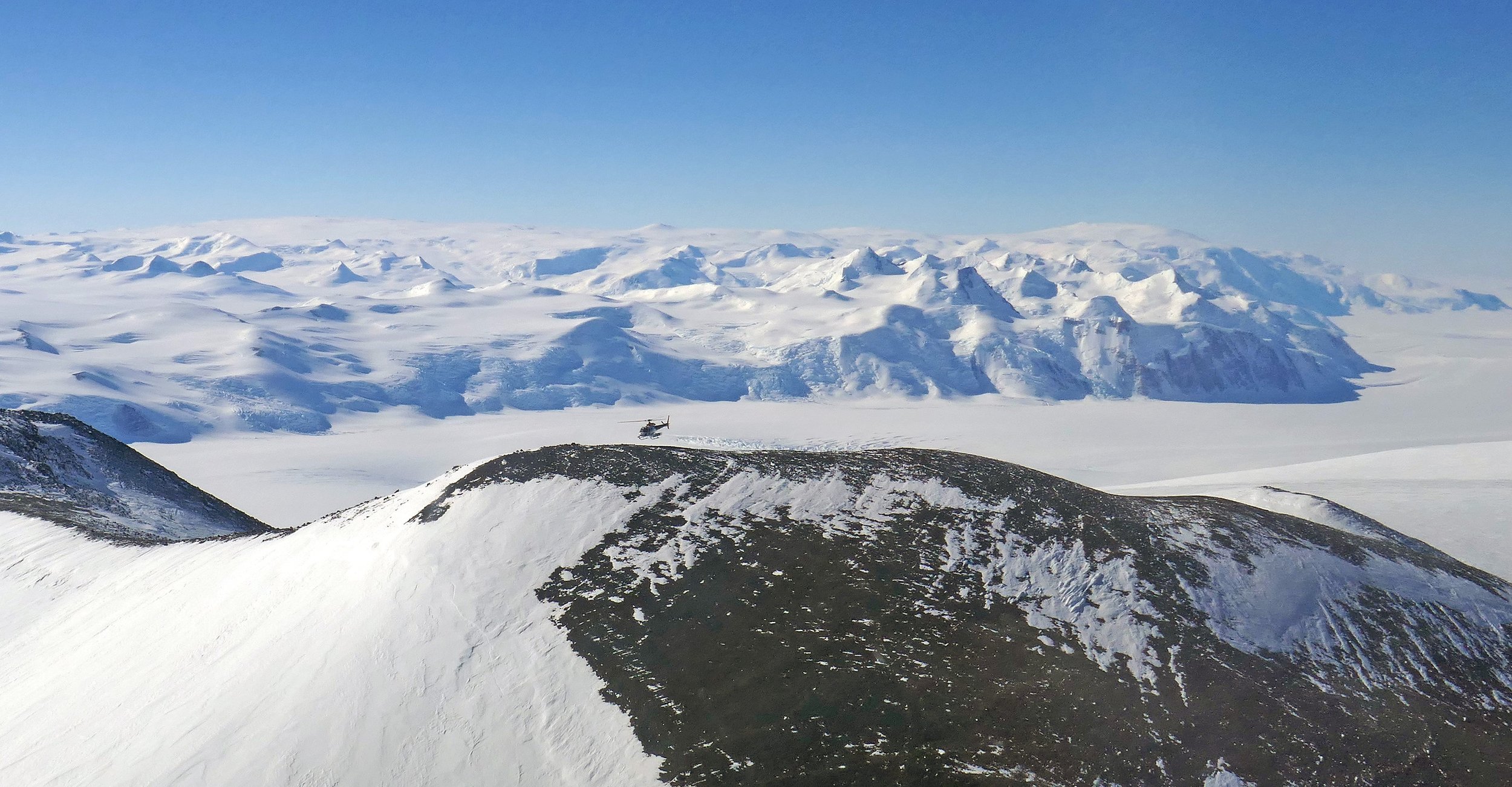
Glaciovolcanism and subglacially erupted volcanoes in Antarctica
Image: A helicopter hovers above the crater rim of a large scoria cone at The Pleiades, northern Victoria Land
Introduction | Ice sheet proxy | Antarctic glaciovolcanism | Antarctic Peninsula Ice Sheet | West Antarctic Ice Sheet | East Antarctic Ice Sheet | Antarctic refugia
Introduction
In common with other environments, volcanoes also erupt beneath ice sheets and glaciers. Examples are well known from currently and formerly glacierized regions of the world, particularly Iceland, British Columbia and Antarctica, but there are also many individual volcanoes with ice-capped summits (e.g. Alaska, South America; even Mt Kilimanjaro in equatorial Africa: 1). The volcanism is distinctive and has been given its own title: ‘glaciovolcanism’. Glaciovolcanism is defined as “the interactions of magma with ice in all its forms, including snow, firn and any meltwater” [2]. It is a young science with a history of sporadic research extending back less than a century but which expanded dramatically after 2000. It now has its own textbook [3]. As well as being physically dangerous (e.g. effects of fallout on towns & cities, farms & farm animals; highly destructive flash floods known as jökulhlaups), eruptions from glaciovolcanoes can also be economically disastrous (e.g. eruption of the ice-capped Eyjafjallajökull volcano in Iceland in 2010, and its financial impact on Europe). Glaciovolcanoes also store uniquely valuable information on past eruptive environments, specifically properties of terrestrial ice sheets, and glaciovolcanic studies have been developed into what is probably now the most powerful methodology for deriving multiple critical parameters of past ice sheets. Although environmental studies have focussed mainly on the Antarctic Ice Sheet [e.g. 4,5,6,7,8,9,10,28], major advances have also been made in characterising the Pleistocene Cordilleran Ice Sheet in British Columbia [12].
Global distribution of glaciovolcanoes. From 52 (modified after 1)
Subglacially erupted volcanoes as an ice sheet proxy
Studies of past terrestrial ice sheets using glaciovolcanic outcrops have been developed extensively, mainly based on numerous investigations in Antarctica, British Columbia and in Iceland, particularly since 2000 (summarized in 3; and see below). Because ice is not preserved in the geological record (it melts), it is not intuitively obvious how volcanic sequences can preserve a detailed record of that ice. However, the following information can be derived routinely from most glaciovolcanic sequences [13]:
i. Was ice formerly present?
ii. What was its age (radioisotopic dating)?
iii. How thick was that ice?
iv. What was its basal thermal regime (a measure of relative ice sheet stability).
v. What was the ice surface elevation?
vi. What was the internal structure of the ice (i.e. relative proportion of snow/firn & ice)?
Glacial sedimentary rocks such as tills are usually our major source of information on former terrestrial ice masses. However, tills are thin (typically just a few metres thick), physically weak and are modified extensively or removed entirely by subsequent ice fluctuations. Weathering following exposure also significantly affects the appearance and properties of tills. By contrast, volcanic sequences are usually thick (often hundreds of metres) and they contain resistant rocks such as lavas and strongly consolidated, hydrothermally altered volcaniclastic rocks. They are thus able to persist through multiple overriding events by ice. Conversely, volcanic eruptions commonly occur at intervals of several tens, to hundreds and even thousands of years. Thus, the volcanic record has a coarse resolution. This is comparable with the record contained by terrestrial glacial sediments but it is generally much worse than in marine sediments. However, the much greater range of ice sheet criteria that can be extracted (see list above), and particularly the ability to date past ice masses precisely (usually by 40Ar/39Ar), makes glaciovolcanic sequences a prime source of information that cannot be obtained any other way. In addition, much of it is quantifiable, unlike for tills.
Prominent glaciovolcano (tuya) in Iceland, a substantial feature erupted under ice. The edifice measures 5 km long and is 600 m high, much too substantial to be completely erased by younger ice sheets, unlike many glacial sediments
Glaciovolcanism in Antarctica
Antarctica is the largest glaciovolcanic province in the world. It is also the longest-lived, covering the period between c. 30 Ma and was present when Antarctica’s ice sheet became established and developed. Antarctic volcanism is also comprehensively described [11]. There are many volcanoes and they occur all the way from the sub-Antarctic South Sandwich Islands, through the Antarctic Peninsula and Marie Byrd Land into East Antarctica, a distance of about 5000 km [14]. They are particularly well developed within the West Antarctic Rift System, one of Earth’s major intracontinental rifts [15]. Eruptions coincided with the development of the Antarctic Ice Sheet. The volcanoes are overwhelmingly mafic but there are also examples of more evolved magmatic compositions [7,16]. They range from very large stratovolcanoes with summit elevations up to 4 km a.s.l. and basal diameters of 40-60 km, to volcanic fields composed of multiple small centres [6,11,17]. Most of the large volcanoes have gentle surface gradients caused by the eruption of multiple lava-fed deltas (the volcanic equivalent of sedimentary deltas), which mimic and thus recreate the profile of any overlying ice coeval with eruptions. This results in volcanoes having shield-like shapes despite technically being stratovolcanoes (i.e. formed of lavas interbedded with thick fragmental deposits; see discussion on nomenclature by Wilch et al. [18]. The individual volcanoes are often extremely beautiful but the extensive cover of snow and ice and their remote locations can make accessing them quite challenging. They are amongst the least visited volcanoes on Earth, particularly those in Marie Byrd Land. However, unlike lower-latitude volcanoes, whose sequences are typically obscured extensively by vegetation, volcanic outcrops in Antarctica are characteristically very clean and often reveal details that are difficult to observe elsewhere. Conversely, many volcanoes (mostly those inland) are largely buried by the Antarctic Ice Sheet and have undergone limited erosion due to protecting cold-based ice [19] making them poor targets for volcanological—environmental studies. By contrast, coastal volcanoes are often very well exposed probably mainly due to marine erosion, and they often contain spectacular cliff sections up to 2 km high and extending tens of kilometres laterally [20].
Map of Antarctica showing the distribution of volcanic centres and location of the West Antarctic Rift System (WARS)
Satellite view of Mount Takahe (Marie Byrd Land), a typical shield-like WARS volcano. Note how little-dissected it is and how it is largely covered by snow & ice
TMA photograph of Mt Cumming (foreground), Mt Hampton & Whitney Peak, three extinct volcanoes in Marie Byrd Land that are almost drowned by the West Antarctic Ice Sheet
Superbly well-exposed Pliocene volcanic rocks, on James Ross Island, illustrating the extraordinary details preserved in many glaciovolcanic outcrops in Antarctica
ANTARCTIC PENINSULA ICE SHEET:
Multiple Oligocene—Miocene glacial and interglacial episodes [King George Island]
King George Island, in the South Shetland Islands, contains evidence for potentially up to four important episodes of ice accumulation together with extensive (& well-dated) volcanic sequences erupted during the intervening interglacials [21,22,23]. However, the supposed evidence for an Eocene glacial on King George Island [24] is very unlikely and only three glacial periods are currently accepted [11]. Two of the glacials are represented by fossiliferous diamicts and marine sediments. Each of the glacials was local in extent but one, the earliest (Polonez Glaciation; the oldest terrestrial record of glaciation in Antarctica), shows indirect but indisputable evidence for the existence of a large ice sheet in East Antarctica that must have extended down to sea level [25]. The ages of the two older glacial episodes are defined mainly by isotopic dating of interbedded volcanic rocks and are 26.64 and 21.8 Ma, corresponding to the Oi2b and Mi1a major glacial episodes, respectively [22]. The age of the youngest glacial episode on King George Island is not well defined due to a lack of dateable materials but is probably < 10 Ma. The associated volcanic sequences are the most extensively exposed interglacial volcanic sequences anywhere in Antarctica. They are now well mapped although many details remain to be published [Smellie, work in progress]. They include no glaciovolcanic rocks but there is indirect evidence for associated ice, probably consisting of relatively small ice caps [22,23 and unpublished].
Stratigraphy of eastern King George Island showing glacial [blue] and interglacial [green] episodes. From 22.
View of well-exposed volcanic & glacial sedimentary sequences on eastern King George Island. From 22.
Geological units exposed in cliffs on eastern King George Island, composed of glacial and interglacial units. From 22.
Interglacial volcanic and sedimentary strata exposed on eastern King George Island, illustrating how detailed published maps now exist for the outcrops. From 22.
Plio-Pleistocene wet-based dynamic ice
The Antarctic Peninsula was the subject of the first modern detailed glaciovolcanic investigations of a past terrestrial ice sheet in Antarctica. It hosts numerous mainly small monogenetic volcanic edifices in volcanic fields with ages extending between 7.5 Ma and present [11,16,17]. Additionally, a few much larger shield-like stratovolcanoes are present in the northern part of the region [38,39]. Of the latter, the longest-lived and environmentally most important by far is the Mt Haddington volcano, which is the largest volcano in Antarctica and dominates the James Ross Island Volcanic Group (JRIVG) [40]. In situ outcrops in the JRIVG extend back to 6.25 Ma, but eruptions may have commenced as early as 10 Myr ago [5]. Although the Mt Haddington summit rises to 1.6 km, it also has a buried volcanic ‘root’ that extends down 5 km [41]. Several of the volcanic outcrops in the Antarctic Peninsula were important for defining features of glaciovolcanism that resulted in fundamental advances for understanding volcano construction as well as for palaeoenvironmental investigations [e.g. 2,4,42,43,44]. Prior to the glaciovolcanic investigations, the morphology and other important characteristics of the terrestrial Antarctic Peninsula Ice Sheet (APIS) were very poorly known for periods prior to the LGM. Yet, like other parts of the Antarctic Ice Sheet, understanding its stability in our warming world is very important because of its influence on global sea levels. In a very real sense, it can act as a bellwether for global change.
Two generic types of glaciovolcanic sequences are present in the Peninsula, known as (1) sheet-like sequences, a sequence type defined in Antarctica [42; see also 3,45] and (2) sequences dominated by multiple pahoehoe lava-fed deltas [2,5,44]. The sequences contain a detailed history of ice sheet thicknesses and other related features, which show that the APIS has varied in thickness up to c. 850 m but was typically much less (< 400 m) most of the time in the period between 6.25 Ma to present [5,6]. It thus simply draped rather than drowned the landscape, i.e. it was an icefield. The basal thermal regime was overwhelmingly wet-based (polythermal?), so it was a dynamic ice mass [2,46,47]. Critically, the only combined glaciovolcanic—ice sheet modelling study to have been undertaken showed that the APIS was probably persistent even through the ice-poor periods corresponding to interglacials, although with reduced thickness and extent [also 48]. The robustness of the APIS is probably because the Peninsula is a narrow high landmass sandwiched between two large warm oceanic water masses (SE Pacific Ocean & Weddell Sea). The relatively warm seas provided moisture and thus precipitation, which fell as abundant snow on the intervening high-elevation Peninsula, something that continues today [35].
Distribution of volcanic fields in the Antarctic Peninsula. From 17.
View of Brabant Island, northern Antarctic Peninsula, a heavily eroded shield volcano. Despite the excellent exposure, the photograph illustrates how difficult access to the sequences is.
View of Vega Island, part of the James Ross Island Group. The mesa-like topography is because the islands are formed of multiple pahoehoe lava-fed deltas.
Schematic diagrams illustrating the two major glaciovolcanic sequence types found in the Antarctic Peninsula. A. pahoehoe lava-fed delta; B. sheet-like sequences. From 6.
WEST ANTARCTIC ICE SHEET
Mid—Late Miocene wet-based ice [Mt Murphy, Marie Byrd Land]
Glaciovolcanic studies of the West Antarctic Ice Sheet remain essentially at a reconnaissance level, despite being the target of at least 20 expeditions [18]. A major ongoing reason is the remote and difficult (therefore costly) access to Marie Byrd Land. The principal results have been summarized by Wilch et al. [18]. They suggested that, following the important pioneering studies by LeMasurier [e.g. 29,30], there was substantial evidence for glaciovolcanism as well as eruptions under ice-free subaerial conditions. However they also took the pessimistic view that the local environmental setting, especially the impact of moving ice causing stoss- and lee-side effects on ice surfaces, precluded establishing palaeo-ice-sheet elevations with confidence.
Detailed glaciovolcanic—palaeoenvironmental studies of the Mid Miocene sequences at Mt Murphy (eastern Marie Byrd Land) have demonstrated a particularly nuanced environmental history [unpublished information of the author]. Coastal Mt Murphy is probably the best exposed and most accessible of all the Marie Byrd Land volcanoes. By contrast, most inland volcanoes are largely obscured by the West Antarctic Ice Sheet. The volcanic sequences at Mt Murphy can be divided into two major parts, mainly corresponding to ages of 9-7 Ma and c. 6.5-5 Ma, although much younger rocks are also present [18]. The studies, combined with those by LeMasurier et al. [31], showed that at least 12 glacial and interglacial episodes can be identified and dated with varying confidence at that single locality. Finally, the ice associated with eruptions was wet based and therefore dynamic. Studies are ongoing.
TMA oblique image of Mount Murphy, eastern Marie Byrd Land, showing the geology. The volcano is unusually well exposed because of its coastal location. Basement rocks in purple. Geology by J Smellie (unpublished)
Summary diagram illustrating inferred major environmental events recorded by volcanism in Marie Byrd Land. From 18.
Summary of environmental events recorded at Mount Murphy. From J Smellie, unpublished
EAST ANTARCTIC ICE SHEET
Dramatic Early Miocene environmental changes [southern Transantarctic Mountains]
The two most southerly exposed volcanoes in the world are situated in the southern Transantarctic Mountains just 300 km from South Pole (87°S), at elevations above 2500 m [26]. A third volcanic centre is also present and is even closer to South Pole but is currently smothered underneath 1.5 km of ice; it is known only from volcanic erratics in a moraine and by the strong magnetic signature of the inferred subglacial source edifice [27]. The volcanic centres are small, extinct (Miocene, c. 20-15 Ma; ages still being verified), and all were previously interpreted as glaciovolcanic. However, a new study has shown that the eruptive setting varied from glaciovolcanic to nonglacial (subaerial) [28]. One of the volcanoes erupted under an ice stream, the first of its kind to be described. The glacial conditions included extremely thick ice, much thicker than today and probably capable of drowning the southern Transantarctic Mountains, despite the warmer climatic conditions at the time (much warmer than present). The other volcano erupted under subaerial conditions. Equally interesting for understanding Antarctic environmental evolution is that a large unfrozen lake was associated with the latter, despite its high latitude and elevation. It was extensive - a few kilometres in length and at least 80 m deep - implying that the surrounding Transantarctic Mountains were largely ice free at the time. The dramatic environmental changes indicated by the volcanic sequences are still being assessed but they should have a major impact on our understanding of how future global warming may affect the stability of the East Antarctic Ice Sheet, the largest ice mass on Earth.
Map showing the location of the most southerly volcanoes on Earth, just 300 km from South Pole
Geology and cross section of Mt Early, a small glaciovolcano erupted when the EAIS was much thicker than today. From 28.
Geology of Sheridan Bluff, a small shield volcano erupted in a large pluvial lake. From 28.
Cartoons showing how the Mt Early volcano formed, within a fast-moving Miocene ice stream. From 28.
Late Miocene polythermal ice [Victoria Land]
Glaciovolcanic sequences are also widespread in Victoria Land, particularly along the Hallett Coast in northern Victoria Land, but also in southern Victoria Land, where they include the large and very beautiful snow-clad Mount Erebus [32]. The sequences occur mainly in overlapping large volcanic shields. However, at least one volcanic centre, at Mason Spur, was erupted mainly during interglacial periods [33]. It is also dominated volumetrically by subaerially erupted ignimbrites and is Antarctica’s only-known ignimbrite volcano. The Victoria Land centres are scattered along c. 800 km of the periphery of the present East Antarctic Ice Sheet that faces the Ross Sea [11]. Those in northern Victoria Land and a few in southern Victoria Land have been intensively investigated in recent years, mostly by the author working with geologists of the Italian, American and New Zealand Antarctic programmes. The sequences are mainly Late Miocene in age (c. 12-5 Ma) and are mainly constructed from multiple superimposed glaciovolcanic ‘a‘ā lava-fed deltas [34]. Other sequence types present are minor, comprising felsic sheet-like sequences, pyroclastic cones and at least one glacio-lacustrine sequence [7,8]. They were formed in association with a glacial cover that was typically only a few hundred metres thick (< 300 m). There is only very limited evidence for ice-free conditions, which are not yet well defined environmentally. The observations suggest the presence of a thin persistent Late Miocene icefield draping the pre-Miocene topography in northern Victoria Land for most of the period, although it may have been confluent inland with a thicker East Antarctic Ice Sheet, similar to the situation present today. Counterintuitively, despite the more southerly (therefore colder) and continental flanking location of centres in Victoria Land, the ice was generally thinner there than in the more northerly, warmer Antarctic Peninsula during the same period [35]. The Late Miocene was also much warmer than today but the Transantarctic Mountains hinterland was already uplifted effectively to its current elevation prior to the volcanism and helped the ice sheet to establish and persist. The coeval glacial thermal regime throughout Victoria Land varied geographically and temporally from wet-based and dynamic, to cold-based (frozen to its bed) and relatively stable [8,10]. The variations have been interpreted to be a result of the terrestrial ice sheet being polythermal, i.e. formed of a patchwork mosaic of wet- based and cold-based ice [9]. This interpretation is radically different from orthodox thinking, which suggests that a one-way step-change from wet-based to cold-based ice took place either at c. 14 or c. 2.5 m.y. ago [cf. 36,37]. The impact of global climate change is dominated to a great extent by variations in thermal regime affecting the Antarctic Ice Sheet. This significant shift in the environmental paradigm suggested by glaciovolcanic studies is thus important.
Map showing the distribution of Cenozoic (mainly Mio-Pliocene) volcanism in Victoria Land. See 11 and 35 for details of the numbered volcanic fields.
View of Mason Spur, a prominent volcanic cliff c. 1000 m high in southern Victoria Land. From 33.
Schematic cross section of the Mason Spur volcanic complex showing how the sequence is dominated volumetrically by ignimbrites. From 33.
Typical volcanic sequence in northern Victoria Land and its interpretation. Dominated by superimposed aa lava-fed deltas. From 11.
VOLCANO REFUGIA AND SURVIVAL OF LIFE IN ANTARCTICA
A potentially important spin-off of the glaciovolcanic investigations in Antarctica is the recognition that, despite ice sheets enduring for millions of years, they were typically thin and were not the persistently much thicker topography-drowning ice masses many modelling studies had suggested. However, at glacial maxima, it is believed that the ice in Antarctica repeatedly swept out to the shelf edge and thus should have destroyed all terrestrial life on the continent [49]. Conversely, evolutionary and biogeographical studies of contemporary Antarctic terrestrial and marine biotas are suggesting that contemporary Antarctic life had ancient origins extending back in time millions of years [50]. It is now recognized that Antarctica’s glaciovolcanoes might have provided crucial ice-free locations as refugia for plants and animals [51]. The refugia consisted of moist, warm, protected ice caves linked to fumarolic activity. The causative geothermal heat is linked to cooling magma chambers beneath the volcanoes. Cooling magma chambers and enhanced heat flow typically persist for many tens of thousands of years, which could have provided the stable, long-lived secure sites that enabled terrestrial life in Antarctica to persist through multiple glacial cycles and regenerate by dispersing during interglacials, thus resolving an enigma that previously had been inexplicable.
Diagram of species richness (i.e. numbers & diversity) versus distance from nearest volcanic field. The pronounced positive correlation strongly suggests that the volcanoes played a major part in preserving terrestrial life in Antarctica through multiple glacial cycles. Based on 51.
View of the interior of an ice cave created by volcanothermal heat. The cave is warm, moist and protected, providing an ideal habitat for helping terrestrial life in Antarctica to survive multiple glacial episodes. Image: Nelia Dunbar
References
1. Edwards, B., Kochtitzky, W. and Battersby, S. 2020. Global mapping of future glaciovolcanism. Global and Planetary Change, 195, 103356, doi.org/10.1016/j.gloplacha.2020.103356.
2. Smellie, J.L. 2006. The relative importance of supraglacial versus subglacial meltwater escape in basaltic subglacial tuya eruptions: an important unresolved conundrum. Earth-Science Reviews, 74, 241-268.
3. Smellie, J.L. and Edwards, B.E. 2016. Glaciovolcanism on Earth & Mars. Products, processes and palaeoenvironmental significance. Cambridge University Press, 483 pp.
4. Smellie, J.L. and Skilling, I.P. 1994. Products of subglacial eruptions under different ice thicknesses: two examples from Antarctica. Sedimentary Geology, 91, 115-129.
5. Smellie, J.L., Johnson, J.S., McIntosh, W.C., Esser, R., Gudmundsson, M.T., Hambrey, M.J. and van Wyk de Vries, B. 2008. Six million years of glacial history recorded in the James Ross Island Volcanic Group, Antarctic Peninsula. Palaeogeography, Palaeoclimatology, Palaeoecology, 260, 122-148.
6. Smellie, J.L., Haywood, A.M., Hillenbrand, C-D., Lunt, D.J. and Valdes, P.J. 2009. Nature of the Antarctic Peninsula Ice Sheet during the Pliocene: geological evidence & modelling results compared. Earth-Science Reviews, 94, 79-94.
7. Smellie, J.L., Rocchi, S. and Armienti, P. 2011a. Late Miocene volcanic sequences in northern Victoria Land, Antarctica: products of glaciovolcanic eruptions under different thermal regimes. Bulletin of Volcanology, 73, 1-25.
8. Smellie, J.L., Rocchi, S., Gemelli, M., Di Vincenzo, G. and Armienti, P. 2011b. A thin predominantly cold-based Late Miocene East Antarctic ice sheet inferred from glaciovolcanic sequences in northern Victoria Land, Antarctica. Palaeogeography, Palaeoclimatology, Palaeoecology, 307, 129-149.
9. Smellie, J.L., Rocchi, S., Wilch, T.I., Gemelli, M., Di Vincenzo, G., McIntosh, W., Dunbar, N., Panter, K. and Fargo, A. 2014. Glaciovolcanic evidence for a polythermal Neogene East Antarctic Ice Sheet. Geology, 42, 39-41.
10. Smellie, J.L., Rocchi, S., Johnson, J.S., Di Vincenzo, G. and Schaefer, J.M. 2018. A tuff cone erupted under frozen-bed ice (northern Victoria Land, Antarctica): linking glaciovolcanic and cosmogenic nuclide data for ice sheet reconstructions. Bulletin of Volcanology, 80:12; doi:10.1007/s00445-017-1185-x.
11. Smellie, J.L., Panter, K.S. and Geyer, A. (eds) 2021a. Volcanism in Antarctica: 200 million years of Subduction, Rifting and Continental Break-up. Geological Society, London, Memoirs, 55, 816 pp.
12. Wilson, A.M., Russell, J.K. and Ward, B.C. 2019. Paleo-glacier reconstruction in southwestern British Columbia, Canada: A glaciovolcanic model. Quaternary Science Reviews, 218, 178-188.
13. Smellie, J.L. 2018. Glaciovolcanism – a 21st century proxy for palaeo-ice. In: Menzies, J. and van der Meer, J.J.M. (eds) Past Glacial Environments (sediments, forms and techniques), 2nd edition. Elsevier, Amsterdam, Netherlands, pp. 335-375.
14. Smellie, J.L. 2020. The role of volcanism in the making of Antarctica. In: Oliva, M. and Ruiz-Fernández, J. (eds) Past Antarctica. Paleoclimatology and climate change. Academic Press, London, pp. 69-88.
15. Jordan, T.A., Riley, T.R. and Siddoway, C.S. 2020. The geological history and evolution of West Antarctica. Nature Reviews, 1, 117, doi.org/10.1038/s43017-019-0013-6.
16. LeMasurier, W. E. and J. W. Thomson (eds.) Volcanoes of the Antarctic plate and Southern Oceans. American Geophysical Union, Antarctic Research Series, 48, 487 pp.
17. Smellie, J.L. 1999. Lithostratigraphy of Miocene-Recent, alkaline volcanic fields in the Antarctic Peninsula and eastern Ellsworth Land. Antarctic Science, 11, 362-378.
18. Wilch, T.I., McIntosh, W.C. and Panter, K.S. 2021. Marie Byrd Land I. Volcanology. Geological Society, London, Memoirs, 55, pp. 515–576.
19. Rocchi, S., LeMasurier, W.E. and Di Vincenzo, G. 2006. Oligocene to Holocene erosion and glacial history in Marie Byrd Land, West Antarctica, inferred from exhumation of the Dorrel Rock intrusive complex and from volcano morphologies. Geological Society of America Bulletin, 118, 991-1005.
20. Hamilton, W., 1972. The Hallett Volcanic Province, Antarctica. United States Geological Survey Professional Papers, 456-C, 62 pp.
21. Birkenmajer, K., Soliani, E. and Kawashita, K. 1989. Geochronology of Tertiary glaciations on King George Island, West Antarctica. Bulletin of the Polish Academy of Sciences, Earth Sciences, 37, 27–48.
22. Smellie, J.L., McIntosh, W.C., Whittle, R., Troedson, A. and Hunt, R.J. 2021a. Lithostratigraphy and age of Oligocene—Miocene volcanic and sedimentary sequences on eastern King George Island, South Shetland Islands, Antarctica. Antarctic Science, 33, 502-532.
23. Smellie, J.L., Hunt, R.J., McIntosh, W.C. and Esser, R.P. 2021b. Lithostratigraphy, age and distribution of Eocene volcanic sequences on eastern King George Island, South Shetland Islands, Antarctica. Antarctic Science, 33, 373-401.
24. Birkenmajer, K., Gaździcki, A. and Krajewski, K.P. 2005. First Cenozoic glaciers in West Antarctica. Polish Polar Research, 26, 3–12.
25. Troedson, A.L. and Smellie, J.L. 2002. The Polonez Cove Formation of King George Island, West Antarctica: statigraphy, facies and palaeoenvironmental implications. Sedimentology, 49, 277-301.
26. Stump, E., Sheridan, M.F., Borg, S.G. and Sutter, J.F. 1980. Early Miocene subglacial basalts, East Antarctic ice sheet, and uplift of the Transantarctic Mountains. Science, 207, 757–759.
27. Licht, K.J., Groth, T., Townsend, J.P., Hennessy, A.J., Hemming, S.R., Flood, T.P. and Studinger, M. 2018. Evidence for extending anomalous Miocene volcanism at the edge of the East Antarctic craton. Geophysical Research Letters, 45, 3009–3016.
28. Smellie, J.L. and Panter, K.S. 2021. Lithofacies and eruptive conditions of the southernmost volcanoes in the world (87° S). Bulletin of Volcanology, 83:49, https://doi.org/10.1007/s00445-021-01475-y.
29. LeMasurier, W. E. 1972. Volcanic record of Cenozoic glacial history of Marie Byrd Land. In: Adie, R.J. (ed.) Antarctic Geology and Geophysics. Universitetsforlaget, Oslo, pp. 251-260.
30. LeMasurier, W.E. and Rex, D.C. 1982. Volcanic record of Cenozoic glacial history in Marie Byrd Land and Western Ellsworth Land: Revised chronology and evaluation of tectonic factors. In: Craddock, C. (ed.) Antarctic Geoscience. University of Wisconsin Press, Madison, pp. 725-734.
31. LeMasurier, W.E., Harwood, D.M. and Rex, D.C. 1994. Geology of Mount Murphy Volcano: An 8-m.y. history of interaction between a rift volcano and the West Antarctic Ice Sheet. Geological Society of America Bulletin, 106, 265-280.
32. Smellie, J.L. and Martin, A.P. 2021. Erebus Volcanic Province. Geological Society, London, Memoirs, 55, 415-446.
33. Smellie, J.L., Martin, A.P., Di Vincenzo, G., Townsend, D.B., Heitzler, M.T. and Ruth, D.C.S. 2022. Eruptive history of Mason Spur, a Miocene—Pleistocene polygenetic volcanic complex in southern Victoria Land, West Antarctic Rift System, Antarctica. Bulletin of Volcanology, 84:93, https://doi.org/10.1007/s00445-022-01601-4.
34. Smellie, J.L., Wilch, T. and Rocchi, A. 2013a. Aa lava-fed deltas: a new reference tool in paleoenvironmental research. Geology, 41, 403-406.
35. Smellie, J.L. 2021. Antarctic volcanism – volcanology and palaeoenvironmental overview. Geological Society, London, Memoirs, 55, pp. 19-42.
36. Webb, P.-N., Harwood, D.M., Mabin, M.G.C. and McKelvey, B.C. 1996. A marine and terrestrial Sirius Group succession, middle Beardmore Glacier—Queen Alexandra Range, Transantarctic Mountains, Antarctica. Marine Micropaleontology, 27, 273-297.
37. Lewis, A.R., Marchant, D.R., Ashworth, A.C., Hemming, S.R. and Machlus, M.L. 2007. Major middle Miocene global climate change: Evidence from East Antarctica and the Transantarctic Mountains. Geological Society of America Bulletin, 119, 1449-1461.
38. Nelson, P.H.H., 1975. The James Ross Island Volcanic Group of northeast Graham Land. British Antarctic Survey Scientific Reports, 54, 1–62.
39. Smellie , J.L., McIntosh, W.C. and Esser, R. 2006. Eruptive environment of volcanism on Brabant Island: evidence for thin wet-based ice in northern Antarctic Peninsula during the late Quaternary. Palaeogeography, Palaeoclimatology, Palaeoecology, 231, 233-252.
40. Smellie, J.L., Johnson, J.S. and Nelson, A.E. 2013. Geological map of James Ross Island. 1. James Ross Island Volcanic Group (1:125 000 scale). BAS GEOMAP 2 Series, Sheet 5, British Antarctic Survey, Cambridge, UK. [available to download at: http://nora.nerc.ac.uk/506743/1/BAS%20GEOMAP%202%2C%20sheet%205%20-%20Geological%20map%20of%20James%20Ross%20Island%20-%20I%20-%20James%20Ross%20Island%20volcanic%20group.pdf]
41. Jordan T.A., Ferraccioli, F., Jones, P.C., Smellie, J.L., Ghidella, M. and Corr, H. 2009. Airborne gravity reveals interior of Antarctic volcano. Physics of the Earth & Planetary Interiors, 175, 127-136.
42. Smellie, , J.L., Hole, M.J. and Nell, P.A.R. 1993. Late Miocene valley-confined subglacial volcanism in northern Alexander Island, Antarctic Peninsula. Bulletin of Volcanology, 55, 273-288.
43. Skilling, I. P., 1994. Evolution of an englacial volcano: Brown Bluff, Antarctica. Bulletin of Volcanology, 56, 573-591.
44. Skilling, I. P. 2002. Basaltic pahoehoe lava-fed deltas: large-scale characteristics, clast generation, emplacement processes and environmental discrimination. Geological Society, London, Special Publication, 202, 91-113.
45. Smellie, J.L. 2008. Basaltic subglacial sheet-like sequences: evidence for two types with different implications for the inferred thickness of associated ice. Earth-Science Reviews, 88, 60-88.
46. Hambrey, M.J., Smellie, J.L., Nelson, A.E. and Johnson, J.S. 2008. Late Cenozoic glacier—volcano interaction on James Ross Island and adjacent areas, Antarctic Peninsula region. Bulletin of the American Geological Society, 120, 709-731.
47. Nelson, A.E., Smellie, J.L., Hambrey, M.J., Williams, M., Vautravers, M., Salzman, U., McArthur, J.M. and Regelous, M. 2009. Neogene glacigenic debris flows on James Ross Island, northern Antarctic Peninsula, and their implications for regional climate history. Quaternary Science Reviews, 28, 3138-3160.
48. Salzmann, U., Riding, J.B., Nelson, A.E. and Smellie, J.L. 2011. How likely was a green Antarctic Peninsula during warm Pliocene interglacials? A critical reassessment based on new palynofloras from James Ross Island. Palaeogeography, Palaeoclimatology, Palaeoecology, 309, 73-82.
49. Convey, P., Stevens, M.I., Hodgson, D.A., Smellie, J.L., Hillenbrand, C-D., Barnes, D.K.A., Clarke, A., Pugh, P.J.A., Linse, K. and Cary, S.C. 2009. Exploring biological constraints on the glacial history of Antarctica. Quaternary Science Reviews, 28, 3035-3048.
50. Convey, P., Bowman, V.C., Chown, S.L., Francis, J.E., Fraser, C., Smellie, J.L., Storey, B. and Terauds, A. 2018. Ice-bound Antarctica: biotic consequences of the shift from a temperate to a polar climate. In: Hoorn, C., Perrigo, A. and Antonelli, A. (eds) Mountains, climate and biodiversity. Wiley, Chichester, pp. 355-373.
51. Fraser , C.I., Terauds, A., Smellie, J., Convey, P. and Chown, S.L. 2014. Geothermal activity helps life survive glacial cycles. Proceedings of the National Academy of Sciences, 111, 5634-5639
52. Smellie, J.L. 2022. Sedimentation associated with glaciovolcanism : a review. In: di Capua, A., de Rosa, R., Kereszturi, G., Le Pera, E., Rosi, M. and Watt, S. (eds) Volcanic processes in the sedimentary record: When volcanoes meet the environment. Geological Society, London, Special Publication, 520. doi: 10.1144/SP520-2021-135
Terminology for ice-clad volcanoes. From 52.